Selenoproteins
Selenoproteins are proteins present across all life realms which include at least one residue of a nonstandard amino acid: selenocysteine (U or Sec), considered “the 21st amino acid”. This molecule has a high similarity to cysteine (C or Cys), but it contains a selenium (Se) atom instead of a sulfur one in its structure. Selenium was discovered by the Swedish chemist Jöns Jacob Berzelius in 1817, and it has been identified as an essential micronutrient element for many life forms since 1957. [1] Moreover, Selenium is almost entirely ionised and this gives the property to be an extremely efficient biological catalyst.
In the human genome, 25 genes for selenoproteins have been identified. These proteins are involved in redox reactions: selenoproteins have biological functions in oxidoreductions, redox signaling, antioxidant defense, thyroid hormone metabolism, and immune responses. Therefore, the functions of selenoproteins are very variable [2]. On the downside though, the use of selenocysteine is an expensive process at the metabolic level, but due to its properties, this amino acid is both more reactive and resistant to a situation of permanent oxidation.
Selenoprotein biosynthesis in eukaryotes
The selenocysteine biosynthesis happens on their own tRNA (tRNA[Ser]Sec). Its codon is a UGA, which normally signals translation termination (STOP codon), but in the 0.01% of cases it encodes for selenocysteine. When a ribosome detects an UGA codon, Sec machinery interacts with the translation machinery to augment the coding potential of UGA codons and prevent premature termination [4]. The cellular machinery identifies which UGA should be stopped and which should be selenocysteine through SECIS elements (Sec insertion sequence element), the most important factor. It is a specific cis-acting stem-loop structure, in the 3′ (UTR) of selenoprotein mRNA that works as a platform for RNA-binding proteins. It forms complexes with EFsec and the SECIS binding protein 2 (SBP1). EFsec interacts with SBP2 to recruit tRNA[Ser]Sec, and it mediets insertion of Sec into nascent protein chains in response to the UGA codon [3]. Thus, its function indicates that the UGA before is a selenocysteine and not a STOP codon.
There are other additional SECIS-binding proteins: L30, which constitute a part of the basal Sec insertion machinery and nucleolin, and eIF4a3; regulatory proteins that modulate the synthesis of selenoproteins.
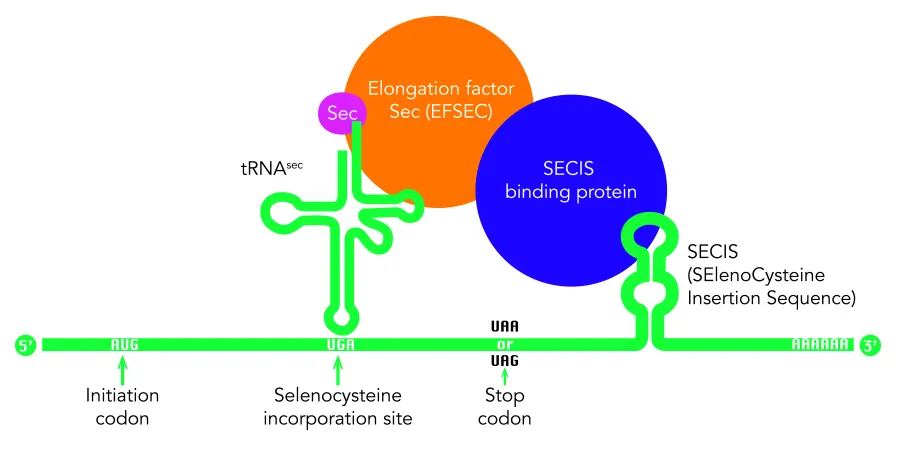
Selenoprotein biosynthesis in eukaryotes
While the genome sequence and gene content are available for an increasing number of organisms, eukaryotic selenoproteins remain poorly characterized. The dual role of the UGA codon confounds the discovery of novel selenoprotein genes [5]. The identification of selenoproteins showed that the mammalian selenoproteome, which was thought to represent most of all eukaryotic selenoproteins, is a small version of the vertebrate selenoproteome. Several selenoproteins were found in lower eukaryotes, and it seems that the environment in which organisms live and the availability of Se may shape selenoprotein evolution. It was seen that there’s reversibility of Sec to Cys replacements. The reason for replacement of selenoproteins with cysteine homologs may be higher oxygen levels, which could have made selenoproteins more susceptible to oxidative damage. For this reason, the highest content of selenoproteins is observed in aquatic organisms. It is thought that the change from aquatic to terrestrial habitats was a challenge to plants and animals, and these organisms were now exposed to higher oxygen levels. Therefore, many terrestrial organisms might have lost selenoproteins or replaced them with cysteine-containing homologs [6].
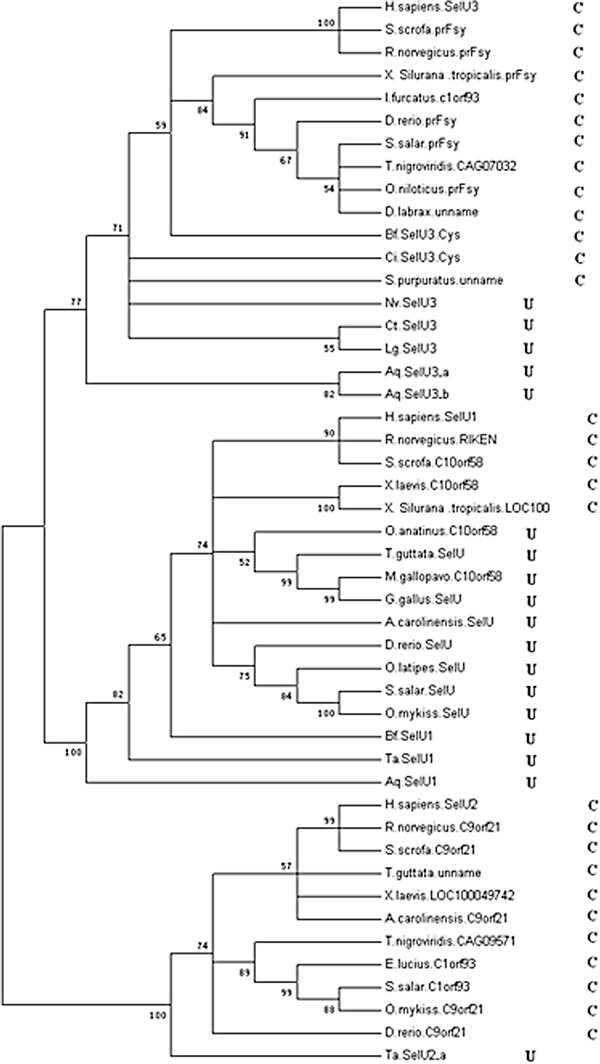